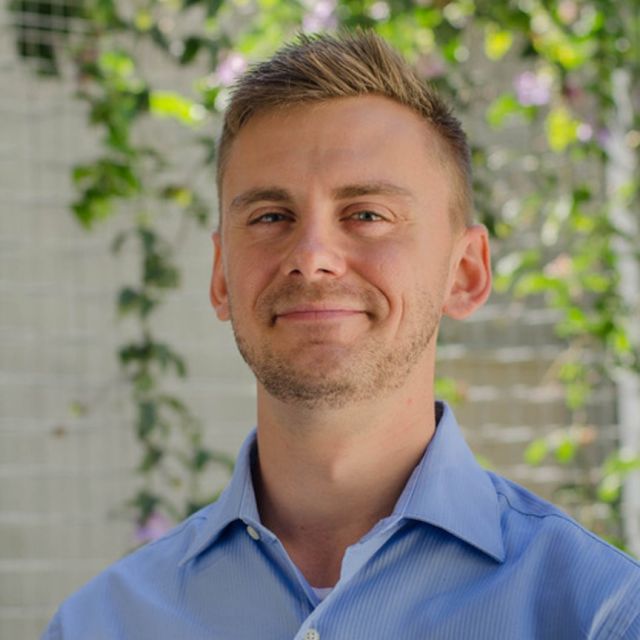
Shaun McKinnie
Intercept and redirect: The role of site-specific vanadium-dependent haloperoxidase enzymology in microbial communities.
The incorporation of halogen atoms in organic molecules plays an important role in modulating their physical properties and biological activities. While the selective chemical addition of halogens can frequently be challenging and employ toxic reagents and byproducts, Nature has developed efficient enzymatic strategies to introduce halides into chemical scaffolds. Site-specific vanadium-dependent haloperoxidases (VHPOs) are a unique halogenase family that employ a histidine-coordinated vanadate ion and co-substrate hydrogen peroxide to oxidize aqueous halides and install them in a regio- and stereospecific manner on organic substrates. Although only ~15 microbial site-specific VHPOs have been extensively biochemically characterized, the vast majority have been involved in the construction of meroterpenoid antibiotics effective against Gram-positive bacterial pathogens. We have identified hundreds of novel VHPO homologs from genomic repositories and are actively exploring many of these genes and their associated biosynthetic gene clusters (BGCs) to isolate bioactive natural products for potential therapeutic applications. Our lab has recently identified a novel family of VHPOs from predominantly Gram-negative bacterial sources which do not appear to be associated with BGCs and with negligible genomic synteny. In vitro biochemical investigation has identified that these VHPOs selectively halogenate alkyl quinolone (AQ) quorum sensing molecules excreted by other microbes, including the notable pathogen Pseudomonas aeruginosa. Halogenation modulates the antimicrobial activities of AQs against both AQ- and VHPO-producing microbes, and this activity is conserved across tens of phylogenetically diverse microbial species. We aim to improve the understanding of this unique family of halogenating enzymes within this concept of microbial chemical ecology and identify its therapeutically relevance. Moreover, we have used this new family of halogenases to develop a model for the mechanism of site-selective halogenation to begin applying this chemistry within non-native chemical scaffolds.
Bio: Dr. McKinnie was born and raised in a rural town in central Alberta, Canada and completed his B. Sc. in Biochemistry (Honors) at the University of Alberta in 2008. He returned to the University of Alberta in 2010 to begin his graduate studies in the Department of Chemistry under the supervision of Prof. John Vederas. His PhD research was supported by the Natural Sciences and Engineering Research Council of Canada (NSERC) and Alberta Innovates Health Solutions (AIHS) and focused on the chemical synthesis and pharmacokinetics of biologically active peptide analogs with improved environmental and proteolytic stabilities. Dr. McKinnie migrated south in 2016 to begin an NSERC-funded postdoctoral fellowship in the lab of Prof. Bradley Moore at the University of California, San Diego and Scripps Institution of Oceanography. His postdoctoral research integrated genomic and biochemical techniques to interrogate how microbes and eukaryotes biosynthesize secondary metabolites with variable bioactivities; these include antibacterial and anticancer small molecules from actinomycete bacteria to neurotoxins from microalgal phytoplankton and macroalgal sources. Since initiating his independent position at the Department of Chemistry and Biochemistry at the University of California, Santa Cruz in 2019, the McKinnie lab uses an interdisciplinary approach to investigate and apply natural product biosynthesis. Through the combination of synthetic organic chemistry, enzyme biochemistry and microbial genomics, our lab explores the role of biosynthetic enzymology in establishing the chemical complexity of bioactive secondary metabolites. In addition to characterizing novel biosynthetic enzymes and their functions, we prioritize key enzymes that establish significant chemical complexity that would be challenging to reproduce in a chemical flask. This allows us to apply enzyme biocatalysis to access larger quantities of bioactive natural products and their analogs without their source organisms for biomedical, pharmaceutical, and industrial applications.